^ Figure 3: Optical Micrograph of the cross-section of the cracks.
Article by Dr. Elaya Perumal Kailasanathan, Corrosion and Metallurgical Consultant, Coimbatore, Tamil Nadu, India
Site information
- Circumferential cracks were found in the middle part, between two baffle positions
- A series of rust spots or scratch lines was present on the outside surface of some tubes
- Some tubes had bends
- No apparent corrosion/rust/pitting on the inside surface
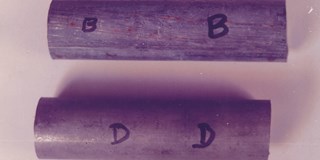
The original preheaters that were installed first started leaking after six years of service. The tubes were suspected to be leaking near the tube-to-tube sheet weld joints. They were plugged and operation continued. Gradually more tubes started leaking and retubing became necessary. The fabrication drawing of the original preheaters showed the following main points: the tubes were of Type 316 stainless steel; the number of baffles was 2; the working temperatures were 200°C (steam) and 170°C (white liquor). The tubes pulled out from the original preheaters showed circumferential cracks in the middle part of the tubes, between baffle positions, without pitting or wall thinning.
Sample testing
Conclusions
Humidity laden with corrosive industrial gases accumulated on these scratches and led to rust formation.
Table 1. Chemical analysis of the samples.
Sample | % Carbon | % Molybdenum |
A | 0.068 | 2.18 |
B | 0.023 | 2.19 |
C | 0.027 | 2.13 |
D | 0.029 | 2.14 |
Cracking
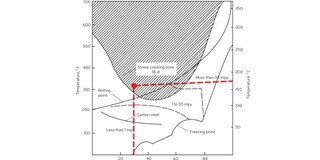
In this case, the corrosion agent was the alkali present in the white liquor. Figure 4 shows a summary of industrial and laboratory data on the caustic stress corrosion cracking of carbon steels and stainless steels. It is clear that with stainless steels cracking occurs at low to high concentrations of caustics at temperatures above 95°C. This environment existed on the tube-side of the preheater.
Table 2. Yield strength and elongation comparison of 316 and 316L.
Material | Yield strength (kg/mm2) | % Elongation |
316 | 36.77 | 46 |
316L | 29.53 | 54 |
Remedial measures
About the author
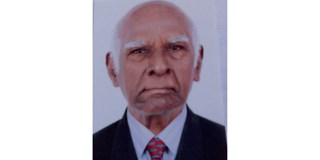